Could supercapacitors disrupt the next generation of electric cars?
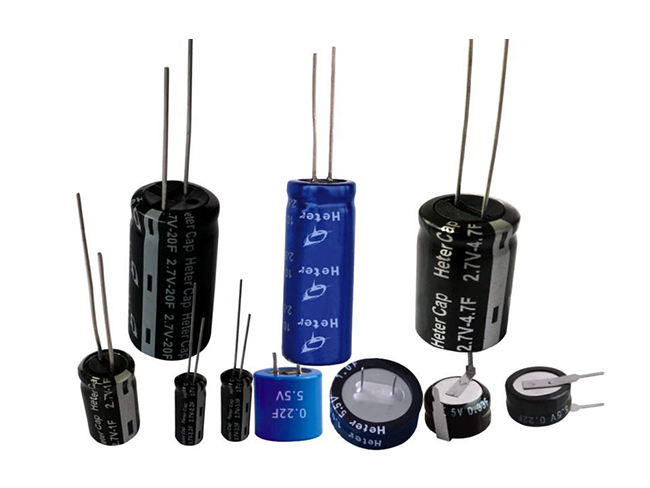
Lithium-ion batteries have played an important role in the development of electric vehicles (EVs). Electric vehicles have become a sustainable means of transportation, but the ultimate vision of electric vehicles is still far away. Engineers and embedded system professionals are looking for better power through supercapacitors. Some experts believe that supercapacitors are a solution that can alleviate the shortcomings of modern batteries, such as service life and charging speed. Is this really the case?
What advantages do supercapacitors bring to electric vehicles?
The efficiency problems of conventional batteries compared to internal combustion engines have led to doubts about the effectiveness of electric vehicles, which is a major problem for the widespread application of electric vehicles. Supercapacitors for electric vehicles are easier to implement than most new technologies, as they are already used in hybrid vehicles. As a result, electric vehicles will gain these advantages, resulting in greater consistency in eco-friendly transportation.
Fast charging and cycle time
The charging time of electric vehicle batteries varies, depending on the amount of charge. However, this is still not as convenient as a gasoline car that can be filled up in a few minutes. Recent studies have demonstrated the ability of supercapacitors to protect against direct surge loads, which can degrade battery performance. At the same time, they can store the recovered energy during regenerative braking, thus improving charging speed and range, which is enhanced by peripheral power applications such as electronic power steering in addition to auxiliary braking.
There have been comments that supercapacitors could have an infinite lifetime if properly designed, but there have also been other suggestions that their lifetime is estimated at one million cycles. The use of intelligent load prediction systems to verify that configurations remain accurate, voltages remain stable, and power levels remain at peak performance saves drivers duplicate battery and maintenance costs, which their high energy storage density also helps to achieve.
Reduce environmental impact
It is well known that lithium-ion batteries and other batteries have a huge environmental impact at the very beginning of their value chain. Although the life cycle of electric vehicles can mitigate their adverse effects, the ecological damage in terms of raw material extraction and energy use is significant.
Supercapacitors for electric vehicles store energy electrically rather than chemically, offering a non-toxic alternative. In addition, the extended service life of supercapacitors improves their life cycle analysis, making them less wasteful at the end of their service life.
Temperature control
Overheating is a concern for lithium-ion batteries, and supercapacitors overcome this negative factor with deeper temperature management. Even under extreme conditions, supercapacitors protect electric vehicle components from performance issues related to temperature fluctuations.
Preventing temperature fluctuations is critical to protecting the energy storage system in the powertrain, which affects other key efficiency metrics. Some new plug-and-play supercapacitors can operate stably between -40 degrees Celsius and 65 degrees Celsius, and can even operate at higher temperatures. In addition, the low resistance of supercapacitors and the lack of chemical reactions make the cooling needs of electric vehicles lower and the cost lower.
Challenges facing the implementation of supercapacitors
Supercapacitors are not a recent innovation - engineers have been using them for decades in industrial, energy and even wearable technology. If supercapacitors offer so many possibilities for electric vehicle development, then why aren't they the gold standard for electric vehicle manufacturing?
The existing electric vehicle problem
In addition to the disadvantages unique to supercapacitors, all the application problems associated with electric vehicles also apply to supercapacitors. Investment, development and attention around new electric vehicle technologies will only begin when there is a general upsurge of interest in electrification. If governments and corporations raise the interest of citizens and commercial entities, more supercapacitor applications will follow.
Cost
The cost of building supercapacitor electric vehicles is higher than standard models, and while manufacturers will invest in this, the burden will be passed on to consumers, severely hampering the adoption of supercapacitors.
This has led researchers to examine opportunities for cost savings throughout the vehicle's life cycle. Experts studied the use of supercapacitors to optimize the cost of refueling and charging infrastructure in public transport, and multi-energy management solutions were the answer, ultimately reducing operating costs by 46.48%.
Voltage limitation
Compared with traditional electric vehicles, supercapacitors operate at a lower voltage. If automakers and engineers want to retrofit existing designs with them, they may need to reconfigure or use voltage converters. Given how supercapacitors simplify the architecture of electric vehicles, this retrofit could make the vehicle's structure more complex than it needs to be.
Low energy density and storage capacity
For chemical reasons, lithium-ion batteries can store more energy per unit weight. Although regenerative braking and other power assist features can extend range, low energy density still results in shorter range, and because supercapacitors release energy for short periods of time, their storage capacity is limited.
Therefore, expanding storage capacity is the focus of research. One example is relying on machine learning to design a new type of supercapacitor material that can store four times as much energy as normal materials, so porous carbon could be the next big thing in enhanced capacitors.
Affect the practical application of electric vehicles
Several companies are trying to use supercapacitors in electric vehicles. As these companies expand, they could spark the adoption of supercapacitors across the industry. So who are the most influential companies?
Swiss startup Morand is solving the energy storage puzzle with its innovative hybrid supercapacitor technology, which is ideal for commuter electric cars and e-bikes, can be charged to 98 percent in two minutes, and will strive to match the price of lithium-ion batteries at scale production.
Another collaboration between Japan's Marubeni Corporation and Skeleton Technologies hopes to expand the marketing of supercapacitors to reach a wider audience. The collaboration addresses another obstacle to the spread of supercapacitors, namely access to components. Eventually, their work could make the technology available to automakers in Europe and Asia.
While these supercapacitor developers are still conducting complex research, they are already being adopted in certain models by automakers such as BMW, Audi and Toyota, which have tweaked brake regeneration improvements and hybrid power units to see where progress can be made. Once players in the industry reach an agreement with automakers, the market value of the entire automotive supercapacitor could reach $239.4 million by 2028.
Supercapacitors, the future of electric vehicles
These recent research results push supercapacitors into a new era of electric vehicle applications, showing more possibilities for electric vehicles, and may change the way society thinks about the potential of electric vehicles. Just as lithium-ion is not the end of battery development, supercapacitors should not stop there, and these improvements should inspire engineers and automotive experts to continue looking for more ways to improve electric vehicles for a sustainable future.
The Products You May Be Interested In
![]() |
AISC-0603-R15G-T | FIXED IND 150NH 200MA 1.12 OHM | 7740 More on Order |
![]() |
ATFC-0201HQ-3N0B-T | FIXED IND 3NH 400MA 300 MOHM SMD | 7074 More on Order |
![]() |
ASPI-0504-102M-T | FIXED IND 1MH 150MA 14.4 OHM SMD | 43020 More on Order |
![]() |
AMPMGEA-6.1440T3 | MEMS OSC XO 6.1440MHZ CMOS SMD | 6390 More on Order |
![]() |
AMPMDGC-4.0000T3 | MEMS OSC XO 4.0000MHZ CMOS SMD | 6696 More on Order |
![]() |
AMPMDDB-18.0000T3 | MEMS OSC XO 18.0000MHZ CMOS SMD | 4644 More on Order |
![]() |
AMJMDEL-90.0000T3 | MEMS OSC XO 90.0000MHZ CMOS SMD | 5994 More on Order |
![]() |
AMPMAGD-8.0000T | MEMS OSC XO 8.0000MHZ CMOS SMD | 6300 More on Order |
![]() |
AMPMAGD-33.0000T | MEMS OSC XO 33.0000MHZ CMOS SMD | 3150 More on Order |
![]() |
AMPMGDD-19.6608 | MEMS OSC XO 19.6608MHZ CMOS SMD | 3690 More on Order |
![]() |
ASDMPC-33.3333MHZ-LR-T | MEMS OSC XO 33.3333MHZ CMOS SMD | 2646 More on Order |
![]() |
ABLNO-156.250MHZ | XTAL OSC XO 156.2500MHZ LVCMOS | 6516 More on Order |
![]() |
AX7HCF1-650.0000T | XTAL OSC XO 650.0000MHZ HCSL SMD | 2520 More on Order |
![]() |
AX7PAF2-466.5600C | XTAL OSC XO 466.5600MHZ LVPECL | 4482 More on Order |
![]() |
AX5DAF1-640.0000C | OSC XO 640MHZ 3.3V LVDS | 4140 More on Order |
![]() |
AX5DBF1-622.0800T | OSC XO 622.08MHZ 2.5V LVDS | 4050 More on Order |
![]() |
ASD1-64.000MHZ-LR-T | XTAL OSC XO 64.0000MHZ HCMOS SMD | 8586 More on Order |
![]() |
ASTMHTFL-13.000MHZ-AJ-E-T3 | MEMS OSC XO 13.0000MHZ LVCMOS | 8298 More on Order |
![]() |
ASTMHTD-100.000MHZ-XK-E | MEMS OSC XO 100.0000MHZ LVCMOS | 3436 More on Order |
![]() |
ASEMPC-24.576MHZ-LR-T | MEMS OSC XO 24.5760MHZ CMOS SMD | 6624 More on Order |
![]() |
ABM11W-45.0000MHZ-4-J1Z-T3 | CRYSTAL 45.0000MHZ 4PF SMD | 3042 More on Order |
![]() |
ABM10W-24.5454MHZ-4-J1Z-T3 | CRYSTAL 24.5454MHZ 4PF SMD | 3438 More on Order |
![]() |
ABM8W-22.1184MHZ-8-J1Z-T3 | CRYSTAL 22.1184MHZ 8PF SMD | 6210 More on Order |
![]() |
ABM8W-28.3220MHZ-4-B1U-T3 | CRYSTAL 28.3220MHZ 4PF SMD | 3654 More on Order |